The selection of refractory materials for kilns is a critical aspect of ensuring the efficiency and longevity of high-temperature processes in industries such as ceramics, metallurgy, glass production, and more. Refractories must be capable of withstanding extreme conditions like high temperatures, mechanical stress, and thermal shock while also providing excellent insulation and chemical resistance.
Refractory materials’ performance can be categorized into physical properties and technical properties. Physical properties include volume, density, porosity, compressive strength, thermal expansion and more, which directly influence the material’s technical performance. Technical performance indicators, such as refractoriness, high-temperature structural strength, thermal shock stability, and high-temperature chemical stability, are essential for selecting the right material for a specific kiln application.
This article will explore five key technical performance criteria that should guide the selection of refractory materials for kilns.
1. Refractoriness (Refractory Temperature)
Refractoriness refers to the ability of refractory materials to resist melting under high-temperature conditions. It refers to the temperature at which a refractory material softens to a certain degree when heated. The method for measuring refractoriness involves grinding the refractory material to a particle size of less than 0.2mm, mixing it with dextrin to form an equilateral triangular cone, and heating it under specified conditions until the top of the test cone bends due to the effects of temperature and its own mass. The temperature at which the cone just touches the bottom plane is taken as the refractoriness. Materials with a refractoriness above 1580°C are classified as refractory materials.
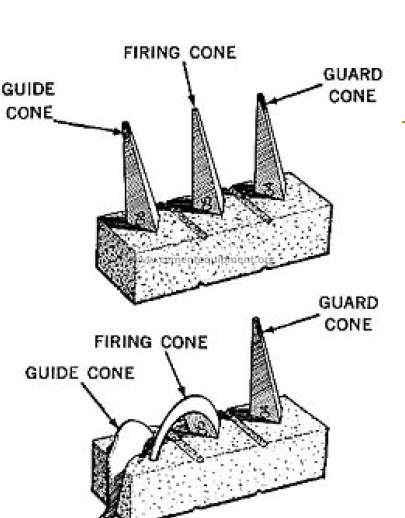
Based on refractoriness, refractory materials can be classified into:
- Ordinary refractories, with a refractoriness of 1580–1770°C, included: fireclay bricks, high-silica bricks, lightweight magnesia refractories
- High-grade refractories, with a refractoriness of 1770–2000°C, for example: high-alumina bricks( Al2O3>60%), chromite bricks, zirconium silicate bricks, magnesite- alumina spinel bricks
- Super-grade refractories, with a refractoriness above 2000°C, for instance: zirconia refractories, silicon carbide, high-purity magnesia, high alumina content refractories, chromium-based refractories(chrome bricks), composite like Silicon Nitride, boron nitride, zirconium boride, titanium boride, molybdenum disilicide, etc.
The refractoriness of a material primarily depends on its chemical composition and the content of easily meltable impurities such as FeO and Na2O. The refractoriness is not the melting point of the material nor its actual working temperature. Since refractories soften under load at high temperatures, the actual operating temperature is lower than the material’s refractoriness.
Importance: Kilns operate at various high temperatures, often exceeding 1000°C, depending on the application. If a refractory material has insufficient refractoriness, it will soften, melt, or lose its integrity when exposed to elevated temperatures.
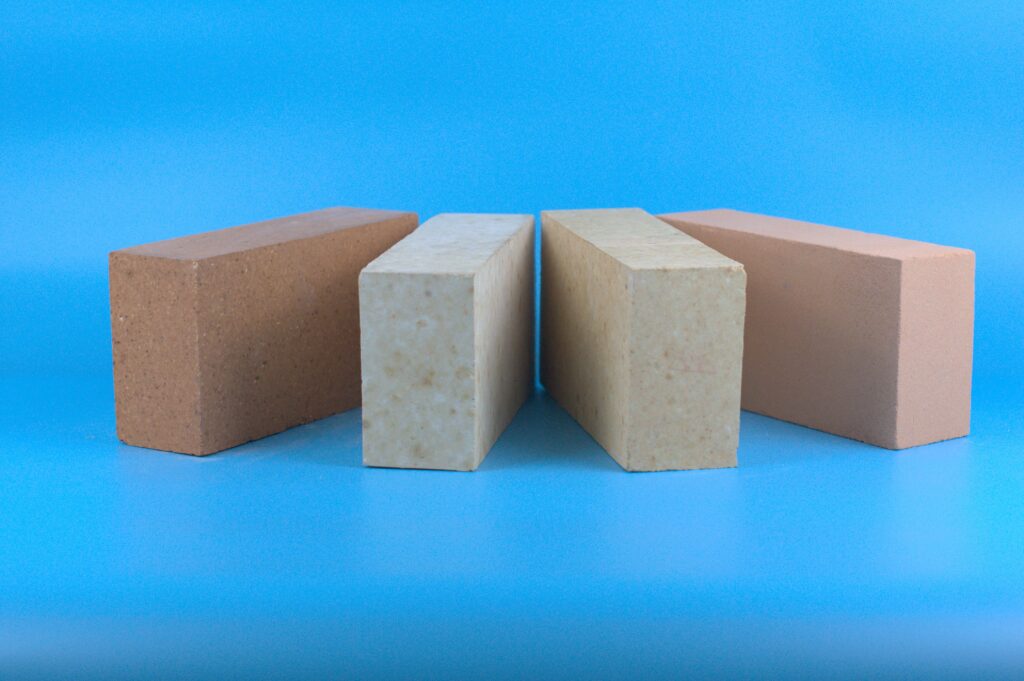
2. High-Temperature Structural Strength
High-temperature structural strength refers to the ability of the refractory material to maintain its mechanical strength and structural integrity when exposed to high temperatures. This property is vital for ensuring that the refractory materials will not deform, crack, or fail under the mechanical stresses encountered during kiln operation.
In practical use, refractory materials must bear certain loads, so high-temperature structural strength (also known as the softening point under load) is an important technical performance index. The softening point under load is commonly used to assess this property. The load softening start point refers to the temperature at which the refractory product begins to deform (by 0.6%) when heated at a certain rate under a specific pressure (196 kPa for heavy materials, 98 kPa for light materials).
Importance: At elevated temperatures, refractory materials are exposed to not only thermal stresses but also physical loads from the weight of products, shelves, and kiln furniture. Refractories need to retain their compressive strength to avoid any risk of structural collapse or failure.
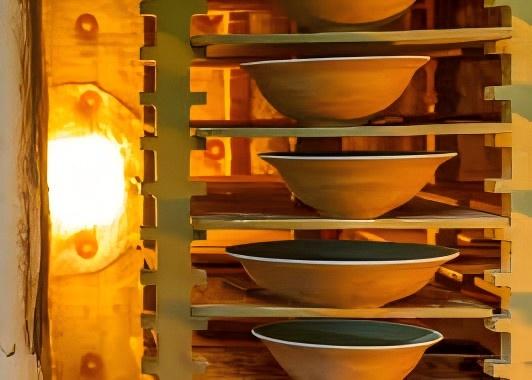
3. Thermal Shock Stability
Thermal shock stability, also known as resistance to rapid heating and cooling, refers to the ability of refractory products to resist damage and spalling when exposed to rapid temperature changes. Thermal shock stability depends on the chemical composition and microstructure of the refractory products. The testing method involves heating heavy refractory materials to 850°C and then cooling them in flowing cold water. This heating and cooling cycle is repeated until the refractory material breaks or loses 20% of its mass, which serves as the thermal shock stability indicator for heavy refractories.
For cyclic operation furnaces that frequently experience rapid temperature changes, if the refractory material does not have sufficient thermal shock stability, it will easily crack or spall or fail prematurely when exposed to thermal stress.
For lightweight refractory products, the thermal shock stability test involves heating standard goods to 1000°C and cooling them in still air, repeating the cycle until the mass loss reaches 20%, at which point the thermal shock stability indicator is determined.
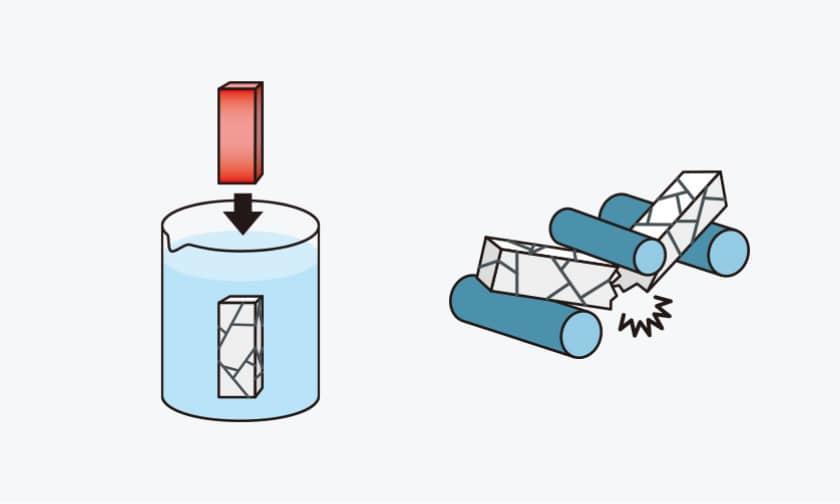
Importance: Thermal shock resistance is crucial for applications with frequent and rapid temperature changes. Without adequate thermal shock resistance, refractory materials are prone to premature failure, leading to unscheduled downtime and increased maintenance costs.
4. High-Temperature Chemical Stability
High-temperature chemical stability refers to the ability of refractory materials to resist corrosion from molten slag, molten salts, metal oxides, and gases in the furnace. This includes both chemical corrosion and physical dissolution, and it is often evaluated by slag resistance. Most evaluations use qualitative indicators. Based on the chemical reactions that occur between the refractory materials and molten slag or salts, refractories can be classified into three categories: acidic, neutral, and basic.
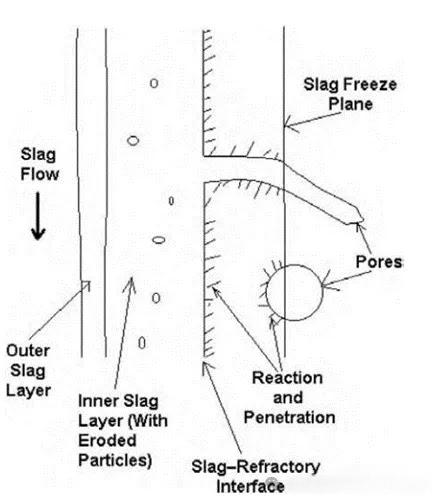
Importance: Refractories exposed to aggressive chemicals—such as acidic or basic slags, metal vapors, or gases—must maintain their structural and chemical stability to ensure safe and efficient operation. Degradation due to chemical reactions can shorten the lifespan of the kiln and increase the risk of product contamination.
Material Selection: High-temperature chemical stability is closely related to the chemical properties and physical structure of the materials.
Alumina, silicon carbide, and zirconia are well-known for their excellent chemical resistance. For instance, clay bricks and high-alumina bricks exhibit certain resistance to both acidic and basic slags. While silica bricks can only resist acidic slags and cannot resist basic slags. Magnesia-based refractories are used in steel furnaces because of their resistance to basic slags. Zirconia offers excellent chemical stability in environments that involve molten glass or other highly corrosive substances.
When manufacturing carburizing atmosphere heat treatment furnaces, the refractory materials used for furnace lining must be carbon-resistant bricks, typically those with an iron oxide content of less than 1% (by mass), because carburizing atmospheres have a strong destructive effect on ordinary refractory bricks.
5. High-Temperature Volume Stability
High-temperature volume stability refers to the ability of refractory products to maintain their volume and shape during long-term use at high temperatures. Refractory materials undergo continuous changes in chemical composition at high temperatures, resulting in recrystallization or further sintering, which can cause irreversible expansion or contraction, and lead to warping, cracking, or poor sealing. These changes are typically represented by the Coefficient of Thermal Expansion and Shrinkage during sintering.
If the volume change is too large, it will affect the strength of the masonry, and in severe cases, it may cause the masonry to collapse. To prevent this phenomenon, it is generally required that the volume change of various refractory products does not exceed 0.5%–1.0%.
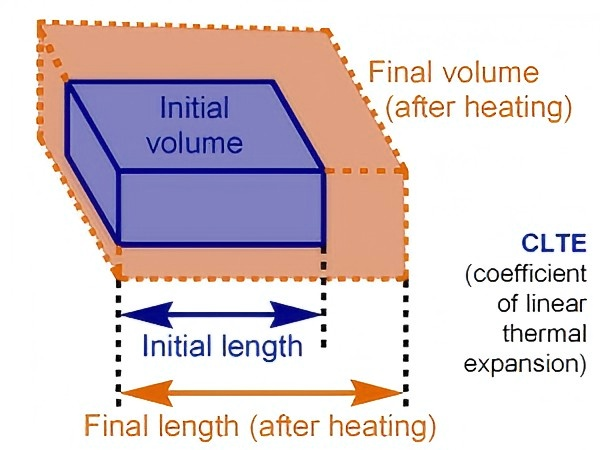
Importance: High-temperature volume stability is particularly important in kilns that operate under continuous or cyclic heating and cooling. Unstable materials can result in dimensional changes, leading to sealing problems, inefficient heat retention, or difficulty in maintaining precise product dimensions.
Conclusion
Choosing the right refractory material for a kiln is a complex task that requires evaluating multiple technical performance criteria. These materials must be able to withstand high temperatures, thermal shocks, and chemical attacks while maintaining mechanical strength and volume stability.
The five key technical performance indicators—refractoriness, high-temperature structural strength, thermal shock stability, high-temperature chemical stability, and high-temperature volume stability—are essential considerations when selecting materials for kilns. By understanding these performance factors, manufacturers can ensure that their kilns operate efficiently, safely, and with minimal maintenance, while optimizing energy consumption and product quality.
In practice, the selection of refractory materials is often a balance between these various performance factors, with different applications requiring different material properties. It is essential to work closely with material suppliers and technical experts to select the best refractory solution tailored to the specific needs of the kiln and its operating conditions.